Contact Info
Adam P. Hitchcock
Canada Research Chair
in Materials Research
CLS-CCRS
B.I.M.R
McMaster University
Hamilton, ON
Canada L8S 4M1
V: +1 905 525-9140
    x24729
F: +1 905 521-2773
E:
U: __________
_____________
  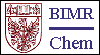
|
What is synchotron light?
Synchrotron light is an accelerator based light source that covers the complete electromagnetic spectrum from infrared to hard X-ray. It is intense, bright, fully tunable, highly polarized, with a time structure useful for many types of dynamics experiments. Aside from the visible, near-IR and near-UV where lasers dominate, the properties of synchrotron light far surpass those of available lab sources.
Historically, synchrotron light was first observed in 1947.
For a long while it was considered just a nuisance to particle physics
experiments since it is the major source of energy loss in all particle
accelerators with greater than ~200 MeV energy. In the 1960's and early
70's pioneering use was made of the light in a parasitic mode - so called
first generation. In the mid-1970's its unique properties and value were
beginning to be appreciated and facilities totally dedicated to synchrotron
light, such as the National Synchrotron Light Source (NSLS) were constructed.
These early dedicated facilities, in which most synchrotron light is produced
at bending magnets, are called second generation sources. In the last
decade new ways of enhancing the yield, and particularly brightness of
synchrotron light were developed. These use high field magnetic structures
placed in straight sections which make wiggles (long period) or undulations
(short period) in the charged particle trajectory which reinforces the
light emitted by the accelerated electrons, much as multiple passes through
a medium with a population inversion is the basis for the special properties
of lasers (see Fig. 1).
A
wiggler generates a quasi continuum, with a flux higher than a bending
magnet roughly in proportion to the number of wiggles. In an undulator
the self-reinforcing aspect of the interaction of the electron beam with
the magnetic structure leads to an N2 gain in brightness, a highly collimated,
partially coherent point source, and a very highly structured energy spectrum.
It is the concentration of all of the emission into the narrow undulator
lines that creates the many orders of magnitude higher brightness of undulators.
The peak energy can be tuned by changing the gap between the magnet poles
- a technological challenge given the forces and precision required, but
one which is fully solved. Synchrotron light facilities which emphasize
insertion devices, and have a high brightness electron beam (small, highly
collimated) are called third generation sources. It is these sources that
are creating tremendous excitement in many areas of science today.
Fig. 2 plots the brightness as a function of photon energy of bending
magnets and various wiggler and undulator devices planned for the Canadian
Light Source (CLS).
Fig.
3 compares average brightness of bending magnet and undulator light from
the Advanced Light Source (ALS) to that of other light sources, such as
an X-ray tube and the sun. The impact of third generation synchrotron
light in the 1990's is conceptually very similar to that of laser light
in the 1970's. At present there is much activity in designing and developing
novel types of insertion devices with properties optimized for special
experiments. At third generation light sources, there is an ongoing dialectic
between the accelerator physics and the synchrotron light experimentation,
since both aspects need to be optimized simultaneously to obtain the best
results. Details of the physics of synchrotron light production [1], and
applications are available in many books [2], journal articles and popular
reports [3].
© 2002 A.P. Hitchcock / McMaster University
- All Rights Reserved
web site by Christopher Amis. Last updated on 04/23/2002 (aph)
|